This book contains exercises for a physical geology lab class. It is under development, with a full 1st edition release planned for Fall 2024.
The goals of this chapter are:
- Understand drainage basins and river patterns
- Interpret meandering rivers using maps and a stream table
- Evaluate hydrographs and flooding
Rivers are an important part of the hydrologic cycle as they provide much of the water that is essential for our existence. They provide food, freshwater, and fertile land for growing crops. While water is essential to life, it can be a destructive force too. When rivers flood, the effects can be catastrophic.They are responsible for the creation of much of the topography that we see around us through erosion and transport of sediment. They are also places of inspiring beauty with magnificent waterfalls, roaring water and in other places slow moving water and tranquility. Mark Twain famously said, “Water taken in moderation cannot hurt anybody.” He was not talking about what happens during large storms and spring snowmelt, when they become raging torrents capable of moving cars and houses and destroying roads and bridges. When they rise over their banks, they may flood large areas, devastating both people and wildlife. In 1931, the Yangtze River in China flooded resulting in second largest death toll for natural disaster with between 1 and 4 million deaths. Experts predict them to become even more severe in the near future as the climate changes.
In an idealized scenario, when a single rain drop falls on the Earth’s surface gravity will drive it (along with other drops) downslope until it reaches a small stream. This stream will meet a river, and this river will flow into more rivers until it reaches a basin, lake or ocean. This means that where the drop falls will determine where it ultimately ends up.
Exercise 12.1 – Discovering Drainage Basins
One great tool for visualizing this process across the globe is River Runner Global. This website allows you to drop a single drop of rain anywhere on Earth, and River Runner will determine which path it will take to the ocean.
Your instructor will choose 6 of the 24 points provided on the map in Figure 12.1 to see where that drop would end up. Drop it as close as possible to the number on the map. For each drop, River Runner will provide statistics and show you the path your drop would take. Do not choose all six points from one region.
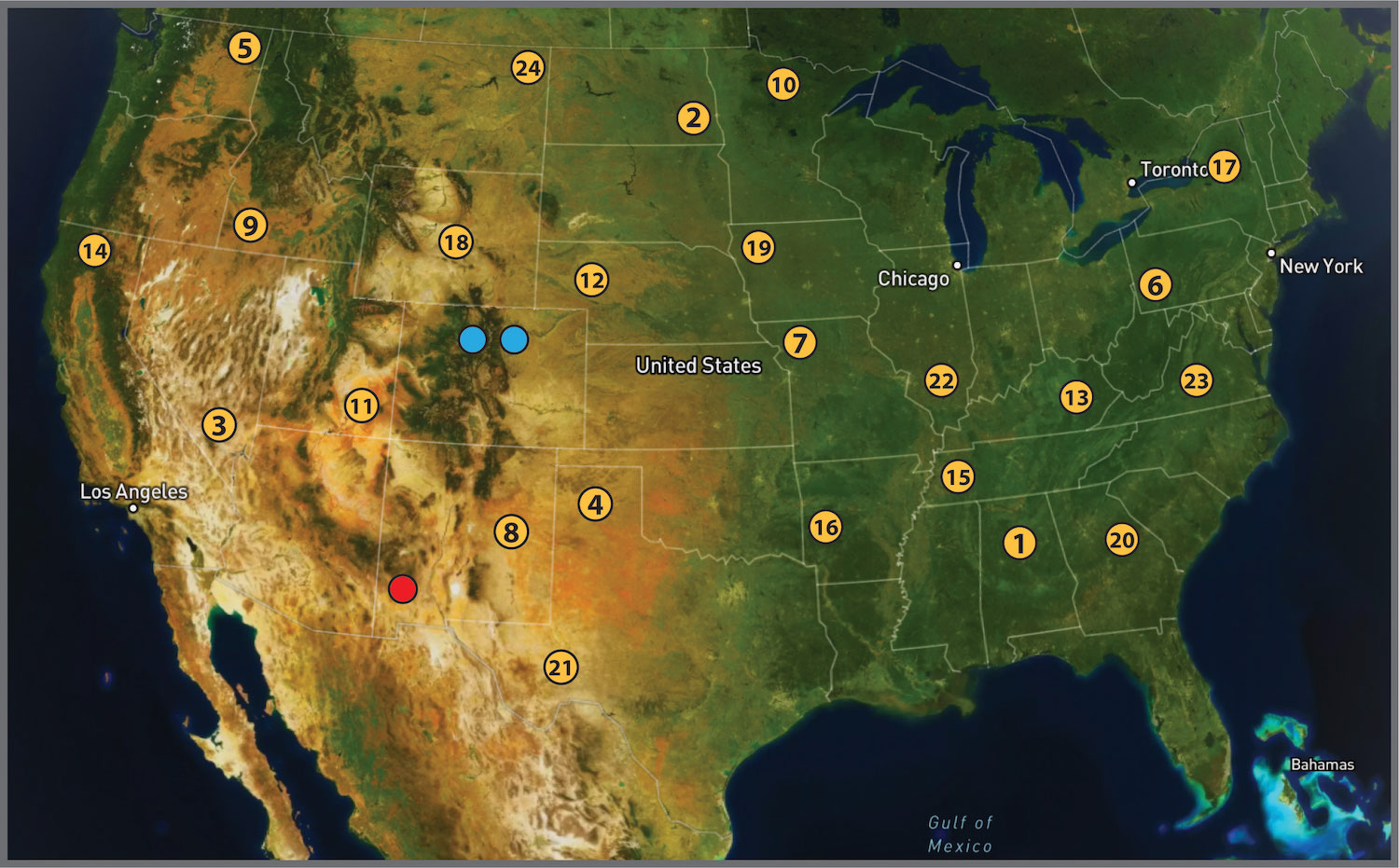
Refer to Figure 12.1 to complete the following questions.
- Before placing any drops, do you think a drop will always end up in the ocean? Why or why not?
- In Table 12.1, record the location number, State, number of rivers (and lakes) the drop passes through, the total distance traveled (km), the river that the drop traveled the longest distance in, and the basin it ended up in.
Table 12.1 – River Runner data No. State Number of Rivers Total Distance (km) Longest River Final Basin - Did you notice any rivers that showed up several times in your paths? Which ones? Why do you think that is?
- Place a drop on each of the blue points in Colorado. In which basins do the two drops end up? Why do you think this is despite them being so close to one another?
- Place a final drop on the red point. Where does it end up? Why?
- Critical Thinking: Why can some drops travel hundreds of kilometers through only one or two rivers, while others travel tens of kilometers through many rivers?
Streams move water continuously from one place to another due to the force of gravity. Streams weather the materials around them and transport it downstream leaving behind channels and valleys. However, many factors can affect how streams shape a landscape. For example, water flowing down a steep slope will move faster than water flowing down a gentler slope, resulting in the steeper slope being eroded more quickly. Or the type of material may influence where a river will flow. This branch of geoscience is called fluvial geomorphology which is the study of the interactions between the physical shapes of rivers, their water and sediment transport processes, and the landforms they create.
Some rivers develop in rocks and others in soils. The shape of rivers is due to an interplay between surface processes, topography, hydrology and geologic structures. Some geoscientists use the geometry of rivers to infer the tectonic history on a variety of scales, from individual fault ruptures to uplift of orogens. An example is the Fraser River in British Columbia, Canada as it follows the Fraser River strike-slip fault zone for 100’s of kilometers.
Exercise 12.2 – Observing Stream Patterns
Instead of following a drop water, observe satellite images of steam networks. Figure 12.2 contains Google Earth Images of four streams with different channel patterns, and Figure 12.3 shows the same streams in black and white.
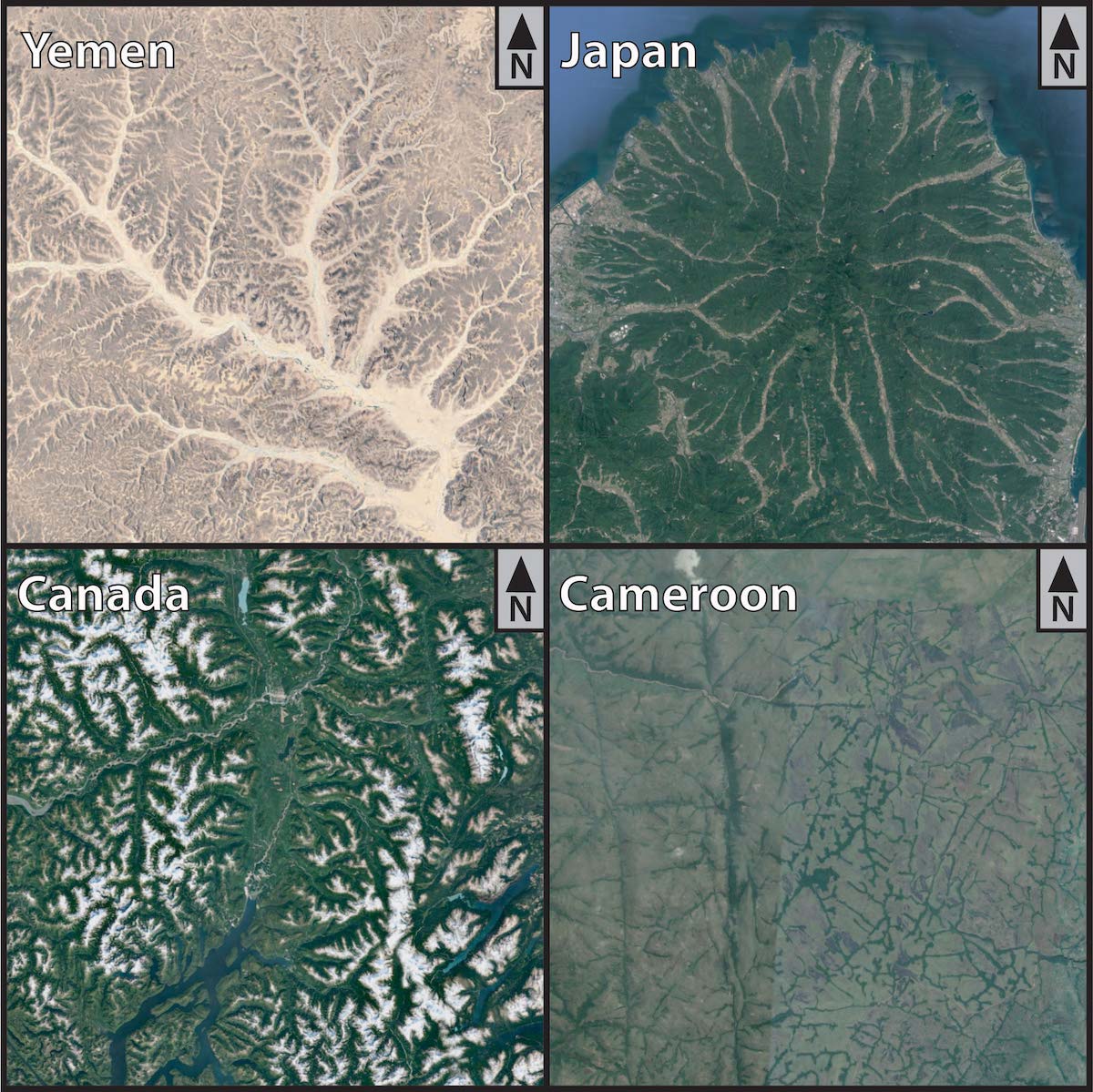
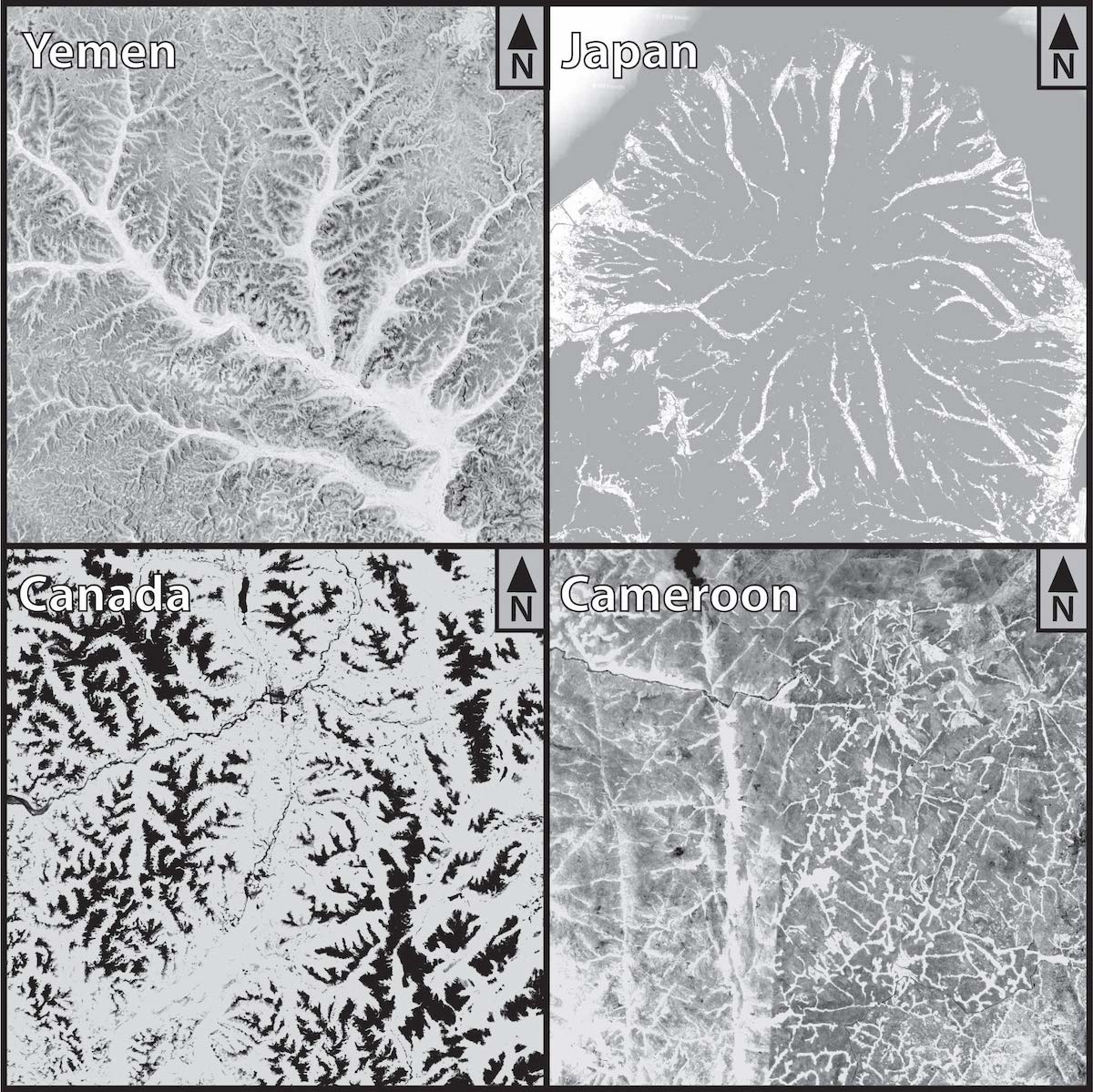
- Look at the patterns for each stream. Trace the patterns in Figure 12.3 and then describe the drainage patterns.
- Yemen:
- Japan:
- Canada:
- Cameroon:
- Yemen:
- What do you think is causing the patterns you observed in each stream?
- Yemen:
- Japan:
- Canada:
- Cameroon:
- Yemen:
- Critical Thinking: Do you think some stream types are more common than others? Why?
As mentioned above, the flow rate in a stream is related to its gradient, but it is also controlled by the geometry of the stream channel. Water flow velocity is decreased by friction along the stream bed, so it is slowest at the bottom and edges and fastest near the surface and in the middle. In fact, the velocity just below the surface is typically higher than right at the surface because of friction between the water and the air. In a curved section of a stream, flow is fastest on the outside and slowest on the inside.
Other factors that affect stream-water velocity are the size of sediments as large particles tend to slow the flow more than small ones and affect the discharge, or volume of water passing a point in a unit of time (e.g., cubic meters (m3) per second). During a flood, as water level rises, there is more area for the water to flow, however, as long as a river remains confined to its channel, the velocity of the water flow also increases.
Rivers can sometimes be seasonal, meaning the channel is dry for part of the year. At other times, they may be flooding; floodplain is an area of flat land next to a river or stream. Rivers can erode their floodplain as they meander, or curve from side to side. Oxbow lakes form when a meander, or bend, in the river is cut off from the river’s main channel. The presence of an oxbow lake documents the meandering river and its tributaries over time.
Sometimes when rivers meet the ocean, they form braided rivers and deltas, where the main channel splits into smaller channels and tiny islands. This can lead to subsidence, in which the elevation of a delta may sink due to sea-level rise or human activity. More about this in the next chapter.
The sediments in floodplains can form aquifers and filter water as it seeps downward. This can create a natural water purification system when water is deliberately filtered through the banks of a floodplain. Nearby wells then collect the filtered water, which is then ready for final purification.
Exercise 12.3 – Understanding Stream Meanders
Meanders (curves in a stream) migrate horizontally across the landscape. As water enters a meander, it flows faster on the outside of the curve and slower on the inside of the curve.
- Figure 12.4 is a Google Earth image of the Ontonagon River in Michigan. On the image, mark where sediment erosion is occurring. These are called cut banks.
- Mark where sediment deposition is occurring. These are called point bars.
- What can you conclude about how a river meander can migrate by erosion and deposition of sediment?
Figure 12.4 – Satellite imagery of the Ontonagon River, Michigan. The river flows eastward. Image credit: Google Earth. - Figure 12.5 shows a section of the Brazos River in Texas. Complete the cross-sections for the different time stamps of the river, showing how the meander migrated and what the sediment would look like. In your cross sections, mark the location of the most substantial erosion with a star ( * ), mark the growth of the point bar with slashes ( \\\ or ///, depending on which way the cut bank was moving), and mark where a lake is filling with laminated sediments with stacked lines ( == ). The first and fifth cross-sections have been completed for you.
- What happens to meanders when they get cut off in Figure 12.5?
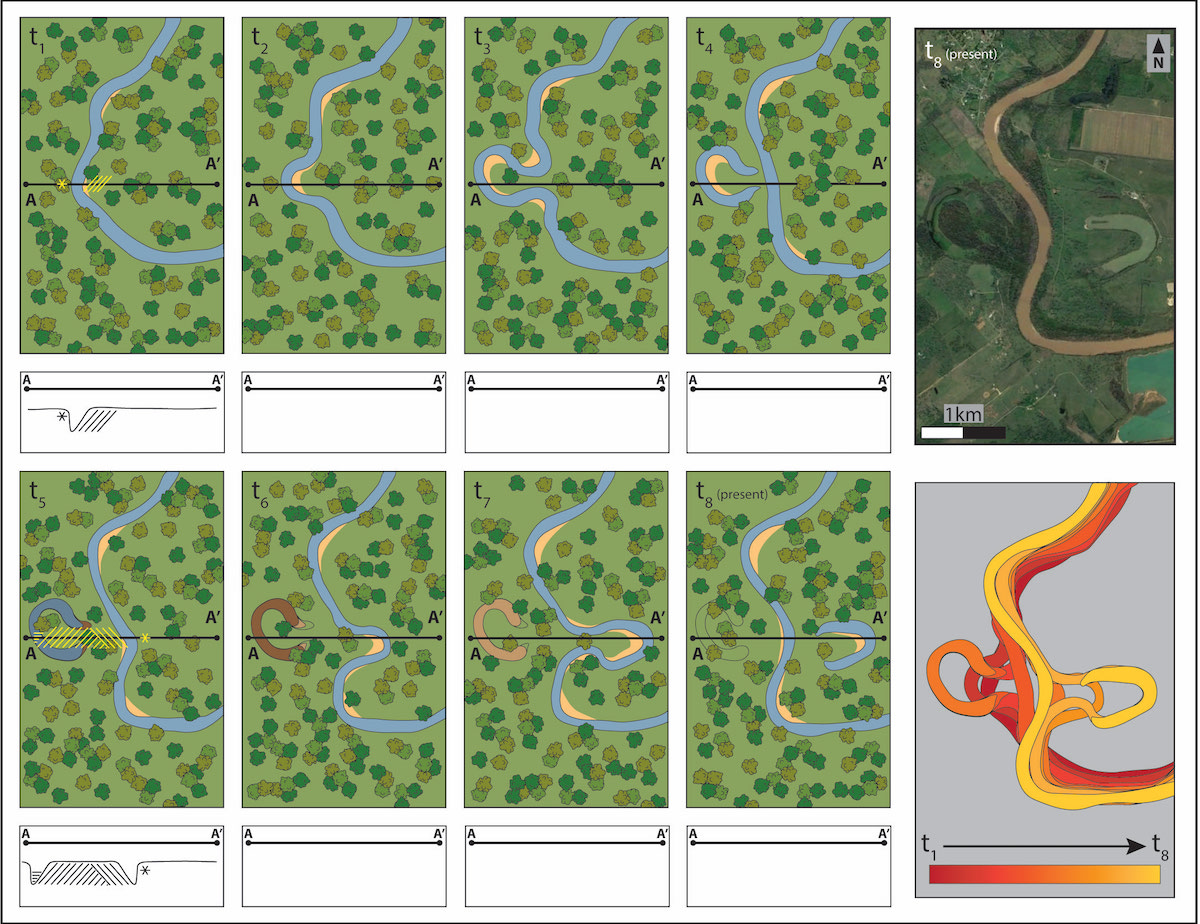
Did you know many borders that separate towns, counties, states, and countries were drawn along rivers? As you’ve learned, rivers move through erosion and deposition of sediment along meanders. While rivers were used as the marking point to separate localities, the official record of most of these boundaries is denoted by latitude and longitude coordinates. So, while the rivers migrate, these boundaries don’t migrate with them. Still, this can cause political issues and border disputes. For example, a land dispute resulted when a river changed its course due to heavy rains some 60 years ago and continues to be unresolved for communities along the Burundi-Rwanda border in central Africa. Another recent dispute in Kittredge, Colorado was resolved in May 2023.
How else do rivers affect people? Well, some floods are seasonal and can be predicted ahead of time. This predictability can make floodplains a good place for urban areas. Rivers provide both a natural transportation network and source of water for irrigation and industry. The relatively flat land can be developed either as agricultural fields or sites for habitation or business.
The three most ancient civilizations on Earth all developed on fertile floodplains. The floodplains between the Tigris and Euphrates rivers, in what are today Syria and Iraq, are known as Mesopotamia, “the land between the rivers.” Ancient civilizations of Mesopotamia include Sumeria, Akkadia, Assyria, and Babylonia. The floodplains of the Indus River, in what is today Afghanistan, India, and Pakistan, gave rise to the Indus River Civilization. Lastly, ancient Egyptian culture developed around the fertile floodplains of the Nile.
Exercise 12.4 – State Lines: A Geopolitical Issue
Figure 12.6 is a Google Earth image of the Mississippi River. The river is over 3,700 km long and defines borders or partial borders for ten states. The section in the image is part of the border between Arkansas and Mississippi.
- Determine the path the Mississippi River took when the state borders were originally drawn in 1836. Some “homes” have been marked on the map for you: blue are in Mississippi and yellow are in Arkansas. Hint: all the blue homes need to be to the right of your line, and all the yellow homes need to be to the left.
- It has been almost 200 years since this boundary was drawn. What does that tell you about the timing of river processes compared to most things in geology?
- Do you think land (and homes) should remain in the possession of a state even if they are isolated by a river in this way? What do you think the landowners would say?
- Explore Google Earth or Google Maps. Can you identify some other borders between localities that would be in a similar situation?
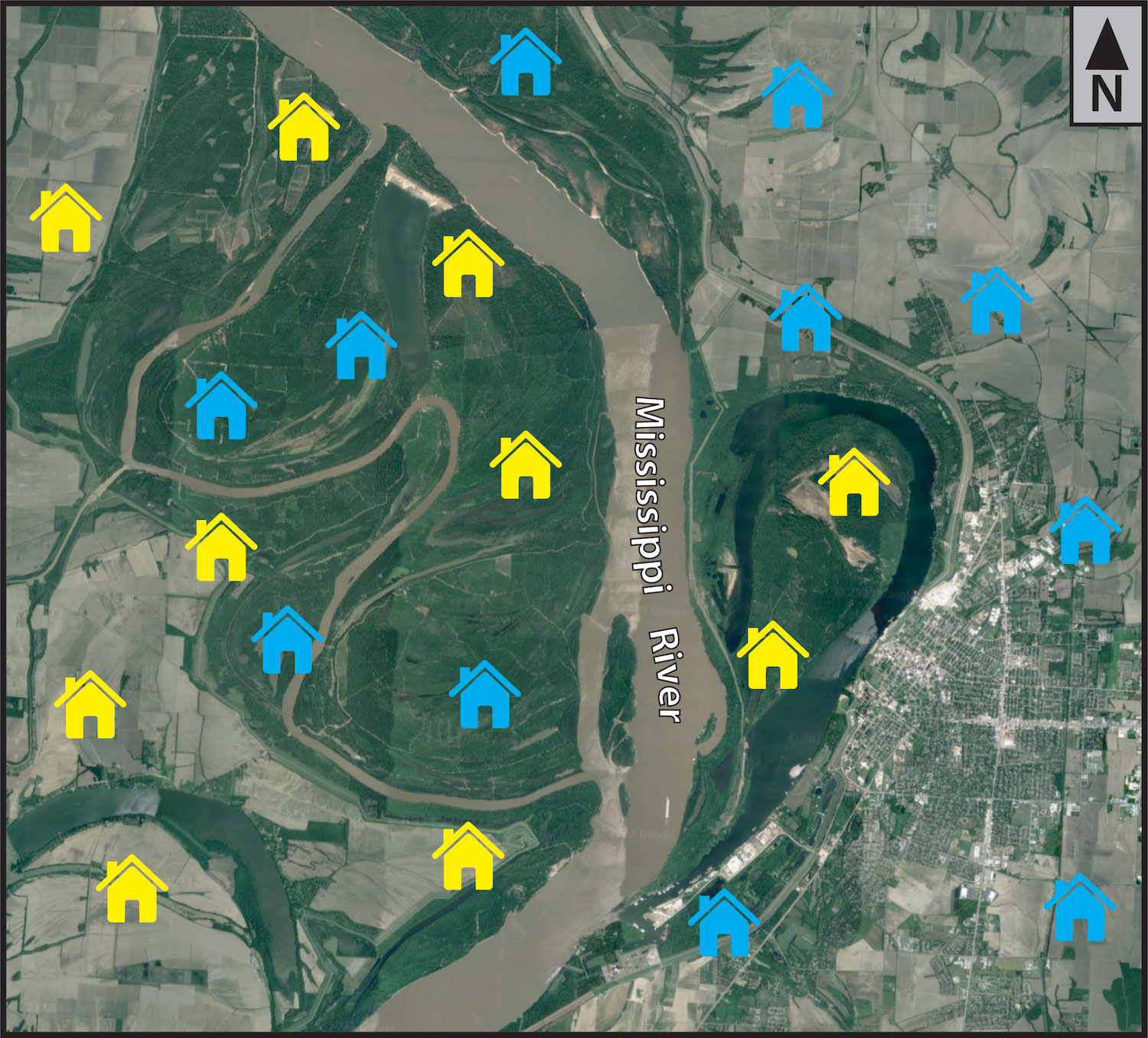
Since it is impossible to experiment with natural rivers, geologists use stream tables with flowing water and moveable media to simulate sediment. River models help us build intuition for processes that otherwise might be hard to visualize. They have the ability to test how a stream erodes, transports, and deposits sediments , which depends on its energy. The higher the energy, the more sediment it can move. Different factors can control the energy level of a stream, including the gradient or slope of the channel. In this lab, we will use modeling to examine the relationship between gradient and different stream types and compare how they erode sediment.
Exercise 12.5 – Modeling Table
Your instructor will take you to the stream modeling table. We have an EM2 from Little River.
The stream channel gradient can be controlled using the standpipe. Pull the standpipe up to lower the gradient and lower the standpipe to increase the gradient. You’ll need to change and measure the height of the standpipe throughout this exercise.
Set up the first scenario and let the water run for a few minutes. Answer a-c. repeat for each scenario.
Scenario A
Lower the standpipe all the way into the table outlet to simulate a steep channel gradient and adjust the stream flow to 75 ml/s.
Scenario B
Raise the standpipe so that the top edge is about 3.5 cm above the outlet. This simulates a moderate channel gradient. Leave the flow rate at 75 ml/s
Scenario C
Raise the standpipe so the top edge is about 7.2 cm above the outlet. This simulates a shallow gradient. Leave the flow rate at 75 ml/s.
- Measure the following characteristics of the streams on Table 12.2
Table 12.2 – Measured stream characteristics Scenario A Scenario B Scenario C Gradient (%) 4 2 0 Channel Length (cm) Straight-Line Length (cm) Sinuosity (channel length/straight length) Valley Shape Valley Width (cm) Channel width (cm) Valley width/channel width - What does the part of the stream channel look like?
- Scenario A
- Scenario B
- Scenario C
- Scenario A
- What does the sediment load look like (low, moderate, or high)?
- Scenario A
- Scenario B
- Scenario C
- Scenario A
- Where is sediment being eroded and deposited?
- Scenario A
- Scenario B
- Scenario C
- Scenario A
- What is the relationship between gradient and stream channel sinuosity?
- What is the relationship between gradient and stream valley shape?
- What is the relationship between sinuosity and the stream valley width to channel width ratio?
- Based on what we know about the relationship between stream channel energy and erosion, what do you think would happen to stream shape and sediment load if we were to increase the stream flow in exercise in Scenario C from 75 ml/s to 150 ml/s? Why?
Flooding is a common natural disaster, and its results are often fatal. As mentioned above, the 1931 China flood occurred when Yangtze and Huai Rivers broke their banks, the flood waters killed many. In addition, the aftermath was devastating with deadly waterborne diseases like dysentery and cholera and those who survived faced the threat of starvation since all the crops were destroyed.
The human cost of flooding can be large, but events like this have a big impact on the natural world too. To help monitor rivers and streams for flooding, the U.S. Geological Survey maintains a network of stream gages to monitor discharge, while the National Weather Service maintains meteorological stations throughout the U.S. If you live near a stream or river, there is a good chance that the water level is monitored as there are over 13,500 stream gauges in the US.
One of the results of a stream gauge is a hydrograph, or a line graph, that depicts water discharge over time for a cross-section within a stream and can show how stream discharge changes following a storm event. Discharge is the volume of water moving through a stream per unit time. In the U.S., discharge is commonly given as cubic feet per second (cfs). A storm hydrograph typically has time on the X-axis and discharge on the Y-axis (Figure 12.7). Sometimes a secondary Y-axis for precipitation is shown. Time typically extends from several hours before a storm event to several hours beyond when the river returns to baseflow conditions. Discharge and precipitation are usually determined remotely using automatic gaging stations.
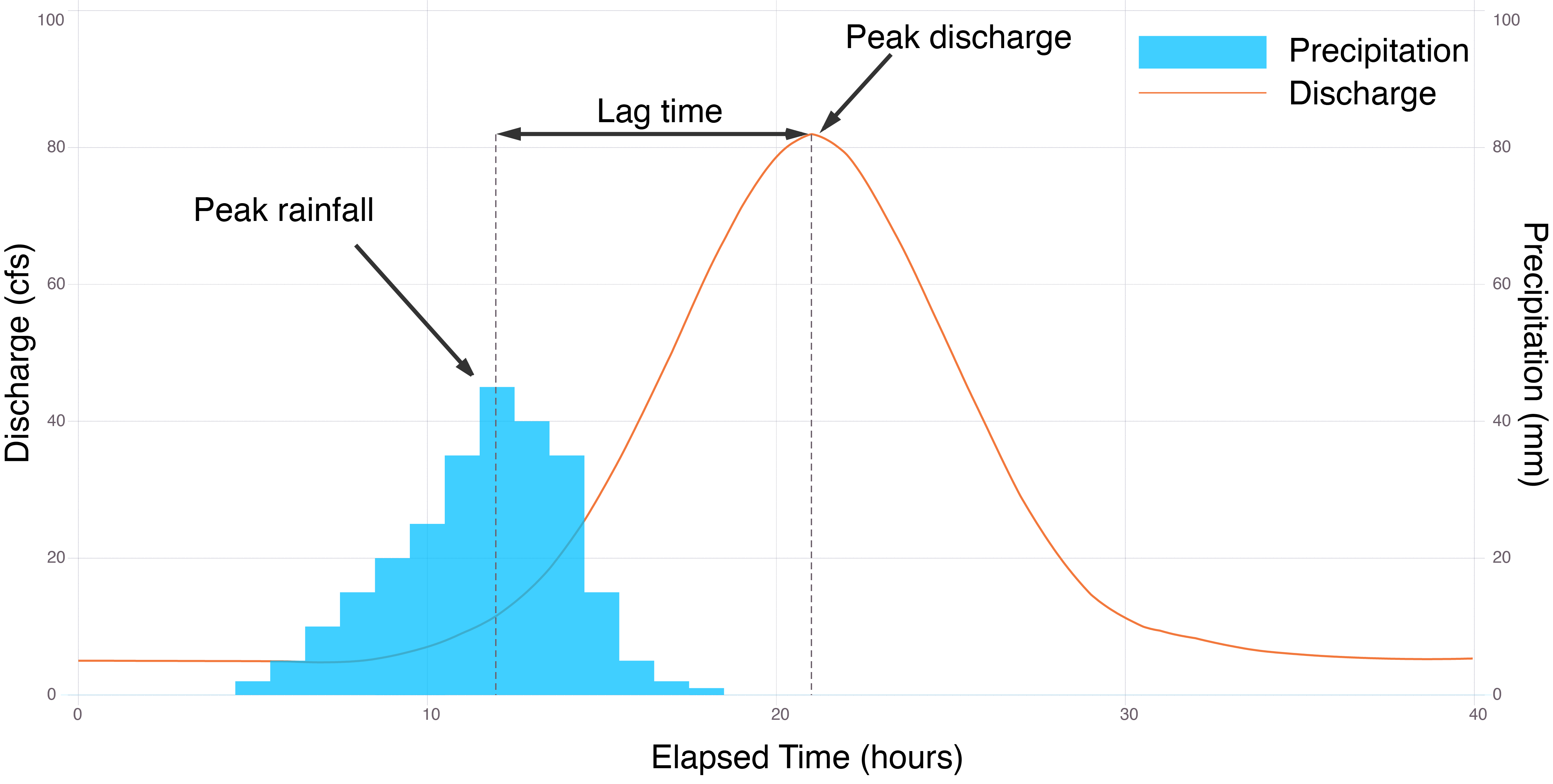
Exercise 12.6 – Create a Hydrograph
Now, let’s investigate some stream gauge data for two different streams.
- Table 12.3 contains precipitation and discharge data for two streams. Plot this data on a single graph. The Y-axes should range from 0 to slightly greater than the maximum values (e.g., if the maximum discharge is 1,382 cfs, the Y-axis range should be 0 – 1,400 cfs). Plot discharge on the Y-axis on the left side of the graph; plot precipitation on the Y-axis on the right side. Use bars for precipitation. You should use different colors for each data set.
- What is the lag time for Station A? ____________________
- What is the lag time for Station B? ____________________
- What is the peak discharge for Station A? ____________________
- What is the peak discharge for Station B? ____________________
- How do the receding limbs on the hydrograph for the two stations compare?
- One of these stations is in metropolitan Houston, while the other is in a rural area outside the city limits. Assuming watershed size is equal, which station is more likely to be the one in Houston (A or B)? How can you tell? Hint: consider how lag times might differ in a city versus a countryside.
Time | Precip. (in) | Station A Discharge (cfs) | Station B Discharge (cfs) |
Noon | 1298 | 765 | |
3:00 PM | 1291 | 768 | |
6:00 PM | 1287 | 756 | |
9:00 PM | 1293 | 791 | |
Midnight | 1297 | 772 | |
3:00 AM | 1299 | 758 | |
6:00 AM | 1295 | 764 | |
9:00 AM | 1297 | 782 | |
Noon | 0.5 | 1376 | 2845 |
3:00 PM | 0.6 | 1456 | 3988 |
6:00 PM | 1.1 | 2245 | 5882 |
9:00 PM | 0.8 | 2578 | 6667 |
Midnight | 0.6 | 2897 | 5058 |
3:00 AM | 3218 | 3982 | |
6:00 AM | 3456 | 2791 | |
9:00 AM | 3877 | 2362 | |
Noon | 4168 | 2023 | |
3:00 PM | 3935 | 1676 | |
6:00 PM | 3812 | 1348 | |
9:00 PM | 3634 | 1201 | |
Midnight | 3502 | 1032 | |
3:00 AM | 3371 | 967 | |
6:00 AM | 3013 | 903 | |
9:00 AM | 2878 | 854 | |
Noon | 2615 | 827 | |
3:00 PM | 2467 | 792 | |
6:00 PM | 2271 | 788 | |
9:00 PM | 2119 | 781 | |
Midnight | 2032 | 783 | |
3:00 AM | 1964 | 789 | |
6:00 AM | 1849 | 782 | |
9:00 AM | 1792 | 778 | |
Noon | 1706 | 780 |
Additional Information
Exercise contributors:
Michael Comas, Virgina Sisson and Daniel Hauptvogel
Reference:
Klepper, MR, Weeks, RA, and Ruppel, ET, 1957, Geology of the southern Elkhorn Mountains, Jefferson and Broadwater Counties, Montana, U.S. Geological Survey Professional Paper 292 doi:10.3133/pp292